Sue
Jinks-Robertson
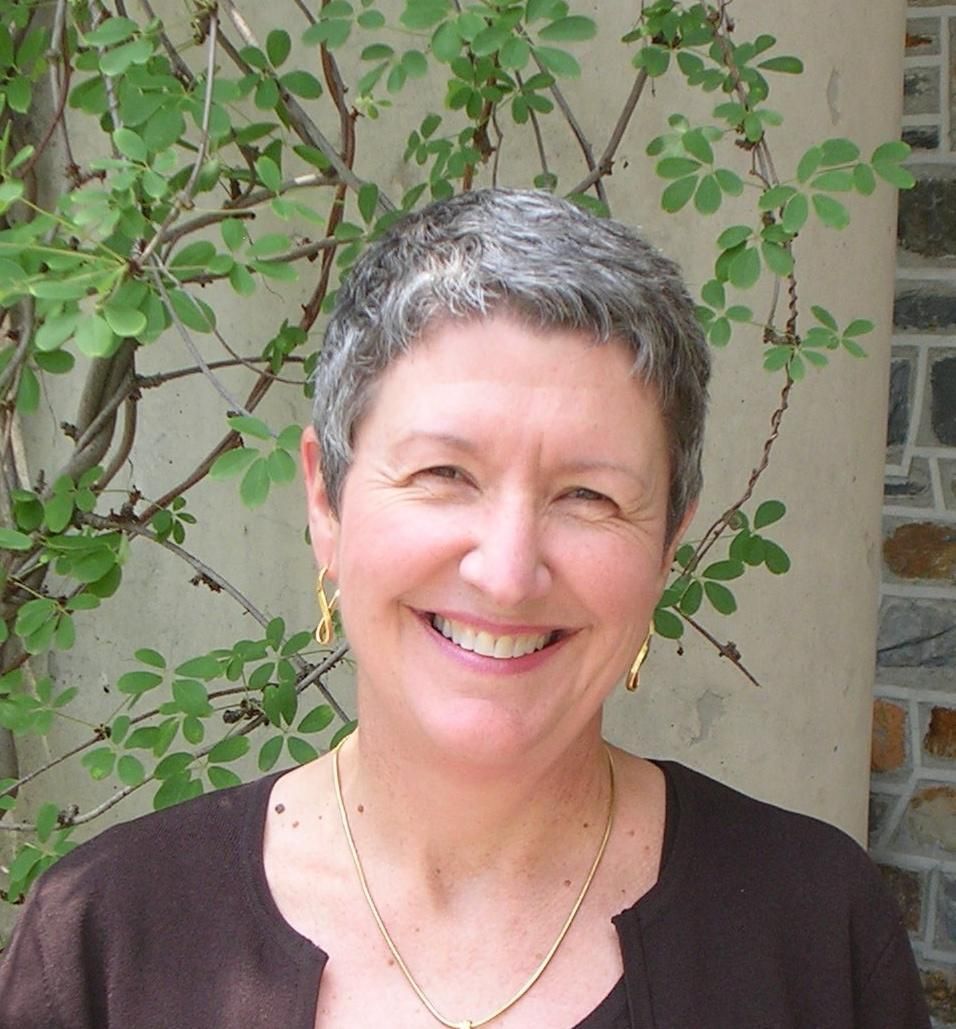
James B. Duke Distinguished Professor Emerita
Professor Emeritus of Molecular Genetics and Microbiology
Member of the Duke Cancer Institute
James B. Duke Distinguished Professor Emerita
384 CARL Box 3020, 213 Research Drive, Durham, NC 27710
Mailing address
384 CARL, Box 3020, Durham, NC 27710